Doctors aren't alone in pioneering health care research. For innovative solutions - page the engineer.
Say “engineer” to most people and they will think of bridges and dams, steam engines and semiconductors, hydroponics and generators, power systems and transportation networks. While infrastructure and machinery are indeed a large part of the work today’s engineers address, what about the delicate infrastructure of the human body? What about the entwined transportation systems propelled by our own blood and plasma? What of the nuanced interactions among cells and synapses, tissues and nerves, inherited genetics and environmental factors? From computer scientists to electrical and chemical engineers, expert researchers at Virginia Tech’s College of Engineering are answering the call to advance health care. Don’t worry, doc. The engineer is in.
Whether using psychedelics to restore mental health or creating fiber optic devices that deliver cancer treatments, the future of effective health care will require continued collaboration between these two fields. Biomedical engineering may be one of the youngest disciplines in the field of engineering, but its potential to improve life on Earth is significant.
Six faculty from the College of Engineering featured below are tackling some of today’s most pressing health-related concerns. These researchers are making vital contributions to biomedical engineering that begin with microscopic cells and extend to predicting and preventing the next pandemic. With the help of Virginia Tech’s world-class facilities, resources, and students, Jenny Munson, Steven Wrenn, T.M. Murali, Padma Rajagopalan, Ryan Senger, and Wei Zhou are tackling medical problems that, like their solutions, are a matter of life and death.
Jenny Munson
Associate professor, Department of Biomedical Engineering and Mechanics, Fralin Biomedical Research Institute at Virginia Tech Carilion
Munson’s research is dedicated to studying the intricate dynamics of fluid movement within the spaces of the brain. Specifically focused on glioblastoma, the most deadly and invasive form of brain cancer, her lab explores how fluid flow facilitates tumor cell migration into the brain, with the end goal of creating tools to enable more effective treatment, both surgical and pharmacological.
In Munson’s words…
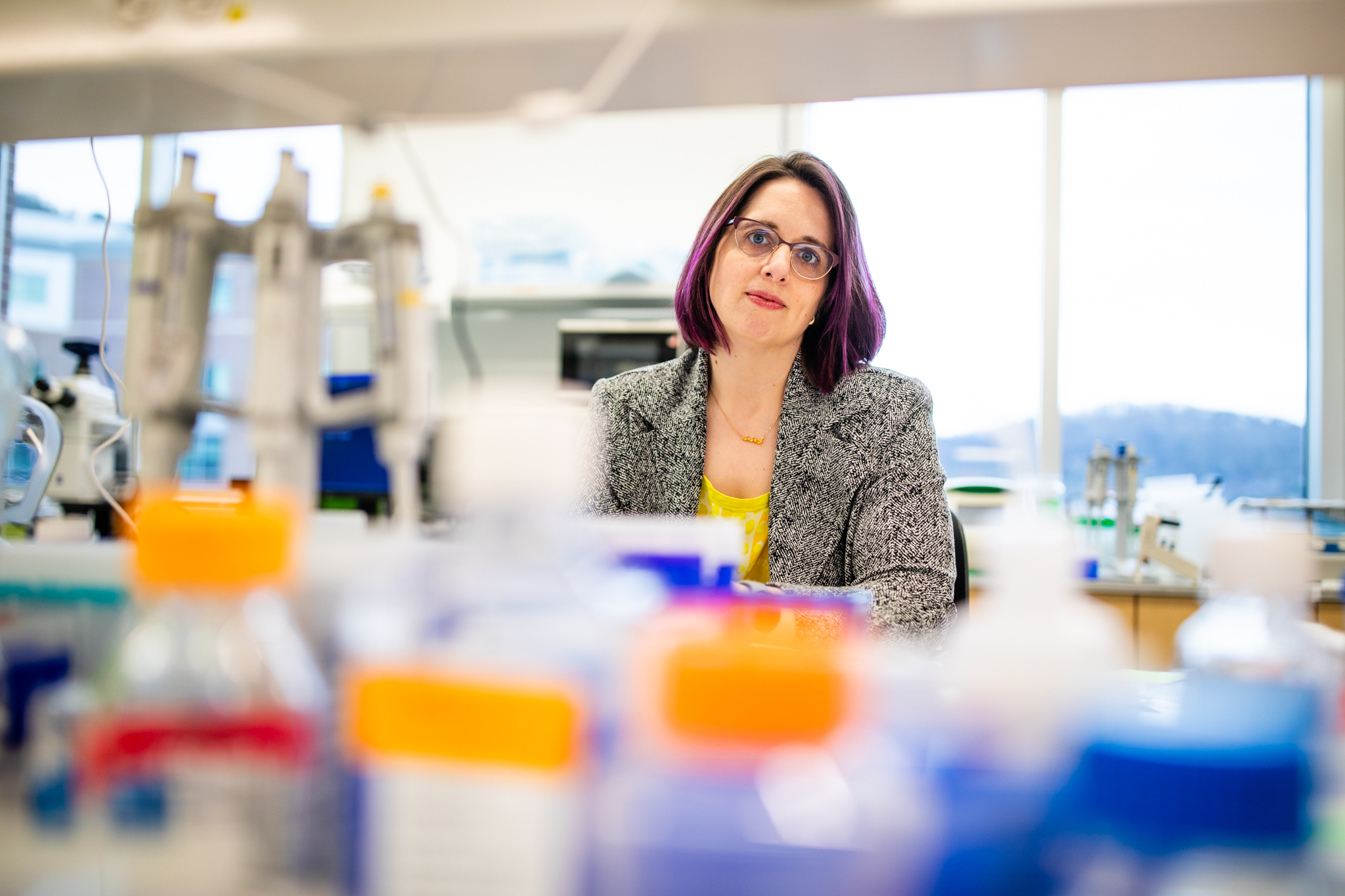
What’s up with the brain?
JM: I'm specifically interested in how fluid moves in the brain. Particularly, the role of interstitial fluid flow (also known as fluid in the spaces of the tissues) in brain cancers. My research looks directly at glioblastoma. We study how fluid flow promotes tumor cells to invade into the brain, which makes them difficult to treat with chemotherapy or radiation therapy or to remove surgically.
Why did the doctor call the engineer?
JM: As engineers, we are problem focused and we're tool builders. For this research specifically, we are building the tools necessary to measure, model, and manipulate fluid flow.
With cancer, we're trying to counteract the effects of fluid flow being too fast. There is a lot we are trying to understand. Our goal is to moderate or regulate the flow of fluid through the brain and there are ways to do that.
One of the methods we use to measure fluid flow in the brain is with data from MRI's that patients already get when they go into the clinic. As a biomedical engineer, my goal is to approach things practically, and by using this technique, we're not having to build something totally new for imaging. We're taking something that's already there, then applying engineering and mathematics to it to extract more information.
My lab builds tools to answer specific questions, which are either clinician informed, literature informed, or even curiosity informed. For example, why does fluid flow change? How do tumor cells invade? To answer these questions, we test using cell culture systems. We've applied tissue engineering to rebuild patient tissues in a petri dish while trying to understand how the flows are changing those tissues. It's always tool building with fluid flow as a core element.
Ideally, in 10 years…
JM: Fluid moving in the body is how things get around. If you deliver a drug, it's going to inherently be linked to how it's being pushed or pulled around in that fluid. If we better understand what the flows and tumors look like, we can guide how or where we target the radiation beam in a tumor, or where we tell a surgeon to cut.
Our research can also tell you if a therapy is ineffective. For example, this treatment isn't getting to the site of the tumor, so we need to try again, or we need to try something different, or design the treatment in a different way. We could be making totally new therapies guiding where we need to go, where we need to treat, to get to the tumor and get it out of there. This could help patients live longer because their tumors won't recur. It could also salvage regions of the brain that don't need to be treated and help with quality of life.
I’d love to see more of a fundamental understanding of how flow varies patient to patient - moving to a more personalized and individual approach to see if we can use flow to understand more about each patient’s case.
Steven Wrenn
Professor and Head of the Department of Chemical Engineering
Wrenn once received a call from a cardiologist asking for help solving a massive clinical problem. The cardiologist wanted Wrenn to develop a novel contrast agent that could be used to diagnose heart disease quickly and inexpensively. Contrast agents are used in medical imaging to enhance the visibility of structures in the body, such as the passage of blood through the walls of the heart using ultrasound. Unfortunately, most contrast agents aren’t very selective. When used, they light up entire chambers of the heart, making it too bright to see what’s happening on the walls. But how to create an agent that would remain relatively dark in the chamber, then light up as it passes through the walls of the heart? Wrenn believes this research highlights the value of chemical engineers in the medical field, demonstrating how engineering skills can provide doctors with new tools allowing them to properly diagnose and treat patients.
In Wrenn’s words…
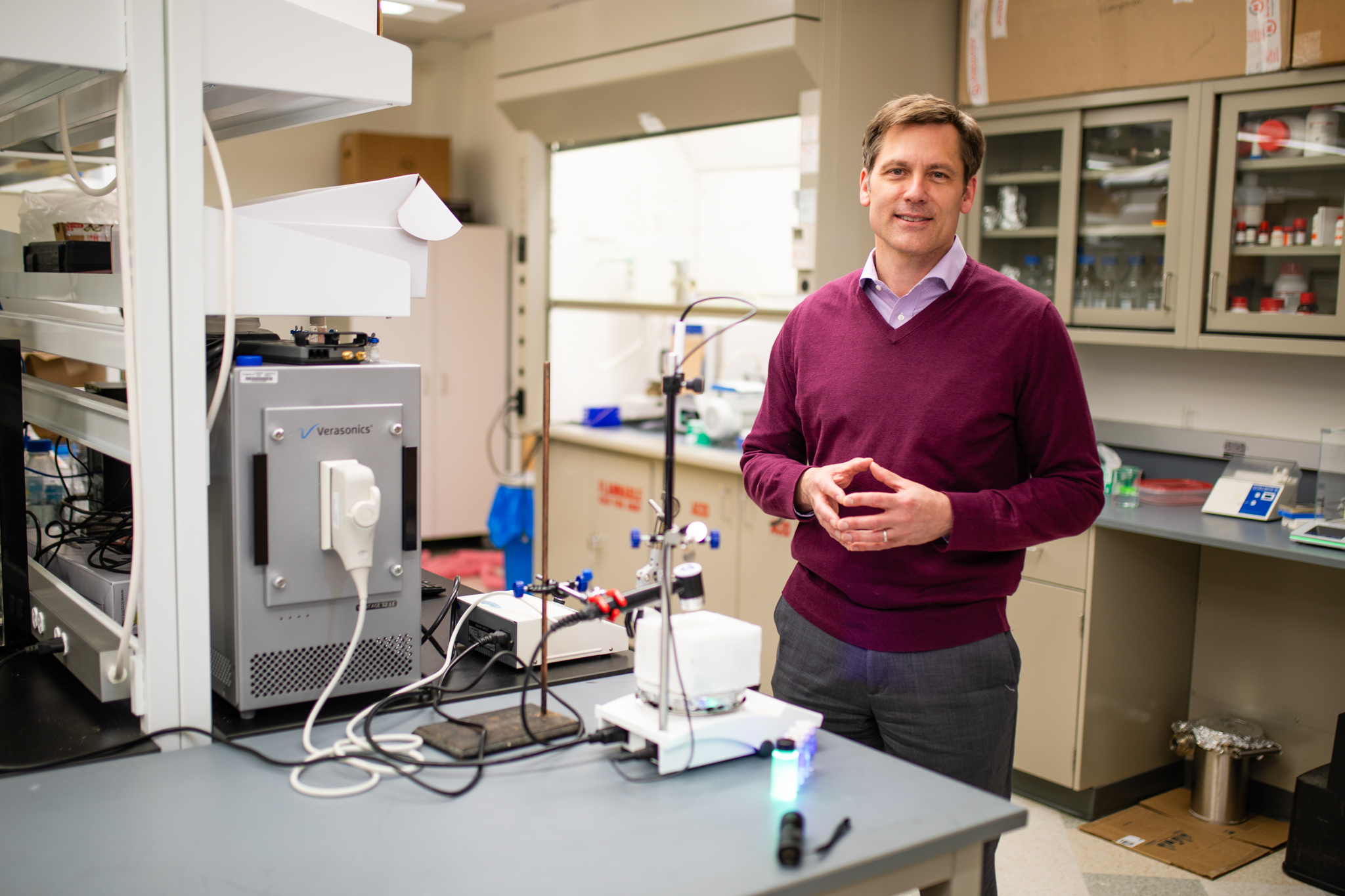
What’s up with the heart?
SW: It is perhaps worth stating the obvious; a doctor cannot see what’s happening inside the heart using only their eyes. If a patient is experiencing chest pain or shortness of breath, the high level question is often whether or not they are having a heart attack. There are current methods that are used to see what’s really going on with the heart, but they can take days, cost thousands of dollars, and are not widely available. These problems led the cardiologist who consulted me to search for what he coined the “Holy Grail of cardiac imaging,” which is to be able to detect perfusion, or the passage of blood through the heart walls using ultrasound. Imaging with ultrasound is ideal because you can use it anywhere and it’s inexpensive and safe. The challenge is that ultrasound by itself cannot see what is happening within the heart walls, and current ultrasound contrast agents brighten the chamber itself more so than the walls. What’s needed is a contrast agent that only becomes active, or lights up, within the walls of the heart. This additional information on the heart would allow doctors to properly diagnose and treat patients.
Why did the doctor call the engineer?
SW: The cardiologist didn’t just say, “We need a contrast agent.” He described the need for a specific agent that could work with the walls of the heart and was sensitive to the environmental differences that exist in the heart walls compared to the chambers, such as different flow rates, pressures, and voltage. He painted the picture of what the ideal solution would be, and then we used our chemical engineering skills to try and create a real version of that. A lot of engineering disciplines can make the claim that most of the products we use in everyday life have to be manufactured. If they can't be grown, they have to be made. It starts with our friends in mining engineering who get the elements out of the ground, our friends, the chemists, telling us how to put the elements together to make a molecule, but then if we're going to convert the molecules into products and do it at a large scale, that's where chemical engineers come into the picture.
Ideally, in 10 years…
SW: We hope there will be a product that satisfies what the cardiologist needs to detect cardiac perfusion using ultrasound. That product’s applications don’t stop there. That same technology, or something very similar, could be used to do targeted, controlled drug release within the bloodstream or inside body tissues so that people have fewer side effects. By leveraging features of the ultrasound agent similar to what provides selective imaging enhancement, doctors can control where a drug needs to go in the body and when it needs to be released, so less medication would need to be prescribed.
T.M. Murali
Professor, Department of Computer Science
Padma Rajagopalan
Professor, Department of Chemical Engineering
More than a decade after they first began bringing chemical engineering and computer science together to tackle critical health care challenges, Padma Rajagopalan, Robert E. Hord Jr Professor of Chemical Engineering, and T. M. Murali, associate department head for research in computer science, have worked together with a faculty team to develop a campus-wide effort to predict and prevent the next global pandemic.
Rajagopalan’s lab focuses on the development of 3D multi-cellular tissue organoids of the liver, gastrointestinal tract, airway, and brain tumors. They design tissue models that explore the physical, mechanical, chemical, and biological environment of a functioning organ.
Murali’s research group focuses on problems in computational systems biology in collaboration with the Virginia-Maryland College of Veterinary Medicine. Currently, they are working on predictive models of viruses that jump from animals to humans (such as rabies or avian flu), host-pathogen interaction and structure prediction, drug synergy prediction, and gene regulatory networks.
To facilitate this and other related work, Rajagopalan and Murali together direct the Computational Tissue Engineering Interdisciplinary Graduate Education Program, and are both affiliated with the Interdisciplinary Ph.D. Program in Genetics, Bioinformatics, and Computational Biology. Their teams are currently collaborating with faculty across Virginia Tech on another high-impact joint research project that goes beyond disciplinary boundaries. This transdisciplinary project, Pandemic Prediction and Prevention (PPP) is focusing on how viruses affect various organs in the body - especially the liver, with which Rajagopalan works extensively in her lab.
In their words…
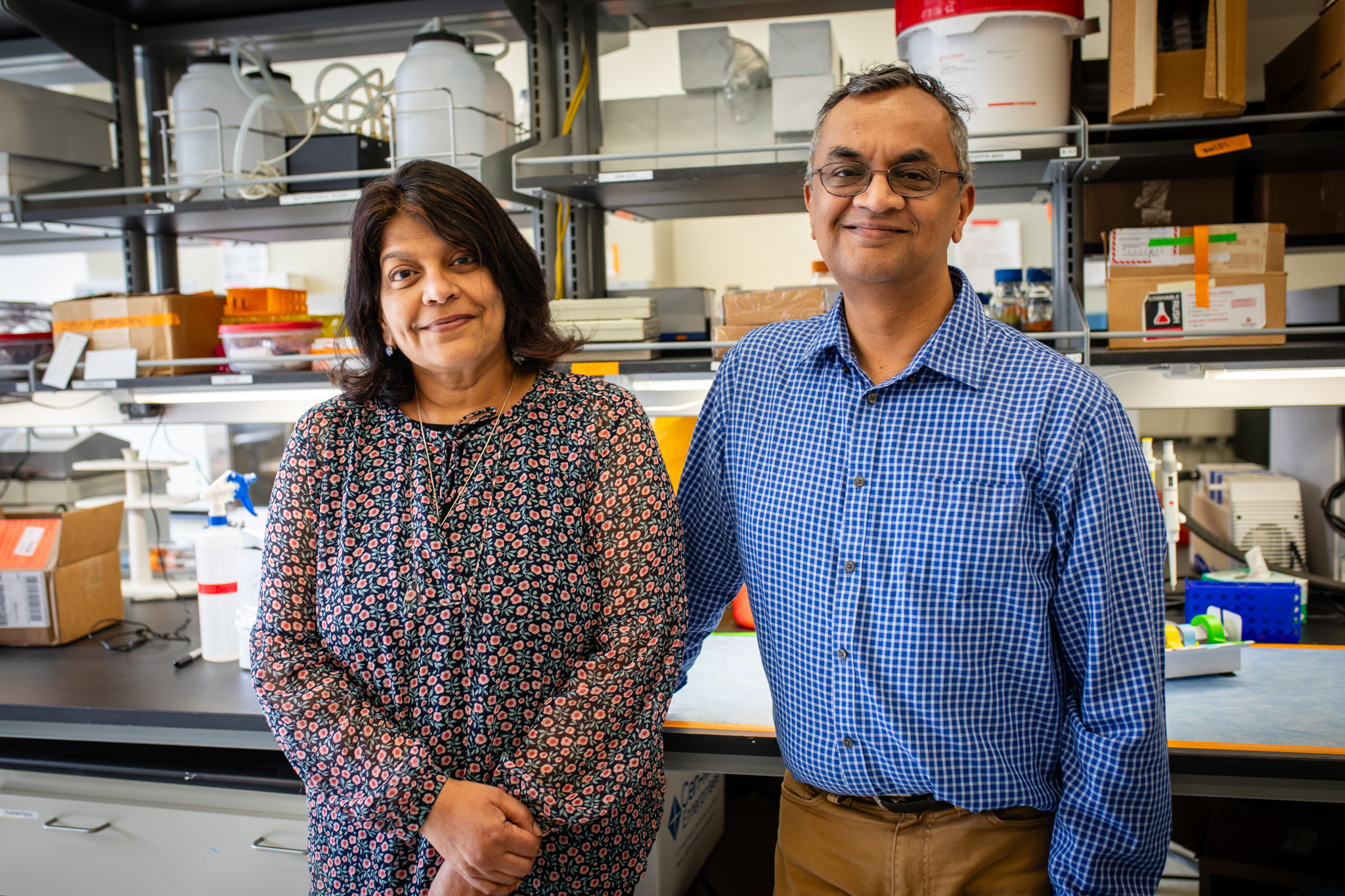
What’s up with the liver, other organs, and viruses?
PR: We design liver models, which are miniature tissue models that mimic key functions of the organ, but we are actually looking at it from new angles. One new angle is applications in understanding how viruses enter, propagate, and potentially cause damage to the liver. Over the years, my lab has designed several different tissue models. Then, of course, there is our virology work through the PPP project.
TMM: Through our project, we want to educate and train a new generation of scientists who have the ability to form teams that can work together, so that we can anticipate future pandemic outbreaks and prevent them from spreading further. And this really can protect and empower humans.
As the COVID-19 pandemic has demonstrated, there are many challenges in trying to determine if a virus can start infecting humans and when it can lead to a pandemic. One of the central challenges is the fact that if you have a new virus - maybe with pandemic potential - it is very hard to grow it in the lab so biologists can study its lifecycle. This is how we begin to understand the virus and develop countermeasures such as drugs or vaccines. Our PPP research group is making the bold claim that organoids are going to play a central role.
Organoids - simplified, miniaturized models of organs that mimic their key functions - will play a central role because with these models we can take a new virus with pandemic potential, grow it in the lab, culture it, and study how it affects the liver or other organs. This will help us develop antiviral drugs, vaccines, therapeutics, and so on, to counter the virus and its impacts on the body.
Why did the doctor call the engineers?
TMM: The work on the liver is very relevant here because one of the viruses we're interested in studying is a type of hepatitis virus called Hepatitis E, which has been found in pork that is sold at grocery stores. There’s a worry that if the meat is not properly cooked so the virus is killed, a human may eat it, and it might potentially spread to us.
It turns out this virus is known to infect humans, but it's very hard to grow in the lab. So we believe Padma's liver models can actually tackle this mystery and enable us to culture and study this virus.
PR: Hepatitis E contributes to a significant number of deaths worldwide. It just doesn't get the same attention that COVID got. As Murali mentioned, it's very difficult to work with it in the lab. So, I've been working with virologists at Vet Med, and together we are using liver models to understand how Hepatitis E infects the liver. We are in the early stages, but we are actually able to show that the virus is infecting the cells. The next step will be to start asking questions like: what is it doing, which parts of a cell does it affect, and what functions does it interrupt?
On the other side of the equation, we can look at testing drugs and therapeutics that could be used against the virus and any complications it causes. I’m collaborating with a professor in chemistry who develops antiviral drugs. It’s important to realize that even something like a flu virus can damage the liver. It may not damage it significantly, but it can compromise liver functions. My collaborator, Webster Santos, a professor in the chemistry department, has developed some new therapies, and we are currently in the process of testing them on our liver models.
One advantage of working with the liver is that virtually every drug and chemical we are exposed to, and every food product we eat, gets processed by it. Because it makes so many different proteins, it does get involved in different infections, so it’s a good place to begin this type of research.
Ideally, in 10 years…
PR: One connection that we've made this past year is with the community. As Murali mentioned, we have public health faculty from Vet Med on our team whom we’ve worked with to hold three different community engagement studio sessions where we talk about different aspects of predicting and preventing pandemics. As part of our project, we want to see what the community’s perception of the science and its possible outcomes looks like. If a therapy comes out of all of these efforts, is that therapy something that the community is willing to use and trust?
PR and TMM: The COVID-19 pandemic has revealed the fact that uncertainty and misinformation in society can reduce trust in science and medicine. Rather than being prescriptive and saying, “Here are the health practices you should bring into your daily lives,” we want to communicate with the public throughout the course of the research and in the future. It's been a really good experience for us because scientists and engineers talk amongst each other a lot. But talking to people from the community who come with very different perspectives has been really helpful, and public health officials have the means to bring people from the community into these discussions.
In our current research, we’ve worked with them to hold three different community engagement studio sessions. This community involvement will be critical whenever we are faced with the next pandemic. We hope that we will be able to succeed in winning a center-scale grant on pandemic science from a federal agency to drive our PPP research forward. Our group will also be recruiting multiple faculty researchers from across the university. We look forward to collaborating with them to make Virginia Tech a nexus of excellence in the pandemic sciences. Finally, we hope to have established trusted bidirectional connections with members of the public.
Ryan Senger
Associate professor, Department of Biological Systems Engineering
Senger conducts research using a technique called Raman spectroscopy, which yields a unique “molecular fingerprint” of complex biological cells, tissues, and fluids. The technique incorporates machine learning, AI, and mathematical tools to interpret different signals returned by laser excitation of a sample, or how the molecules respond to the laser's light. Because individual molecules react differently to laser excitation, complex samples with thousands of different molecules, such as human urine, produce a unique complex Raman signal called a “molecular fingerprint.” These Raman fingerprints are set to identify diseases and can indicate whether a person is dealing with a health issue.
In one application, Senger uses the technique to study human urine and chronic kidney disease (CKD). Typically, CKD is diagnosed through a blood test that measures creatine levels in the blood. This testing can often take a period of time to return results, potentially delaying the start of treatment. Senger is currently working on developing a cost effective urine test that will allow researchers and doctors to further understand the causes of CKD along with potentially earlier detection. With the help of student researchers, and collaborations with Carilion Clinic, the College of Science, and biomedical engineering faculty at Virginia Tech, Senger is continuing to put his research at the forefront of innovative research explorations.
In Senger’s words…
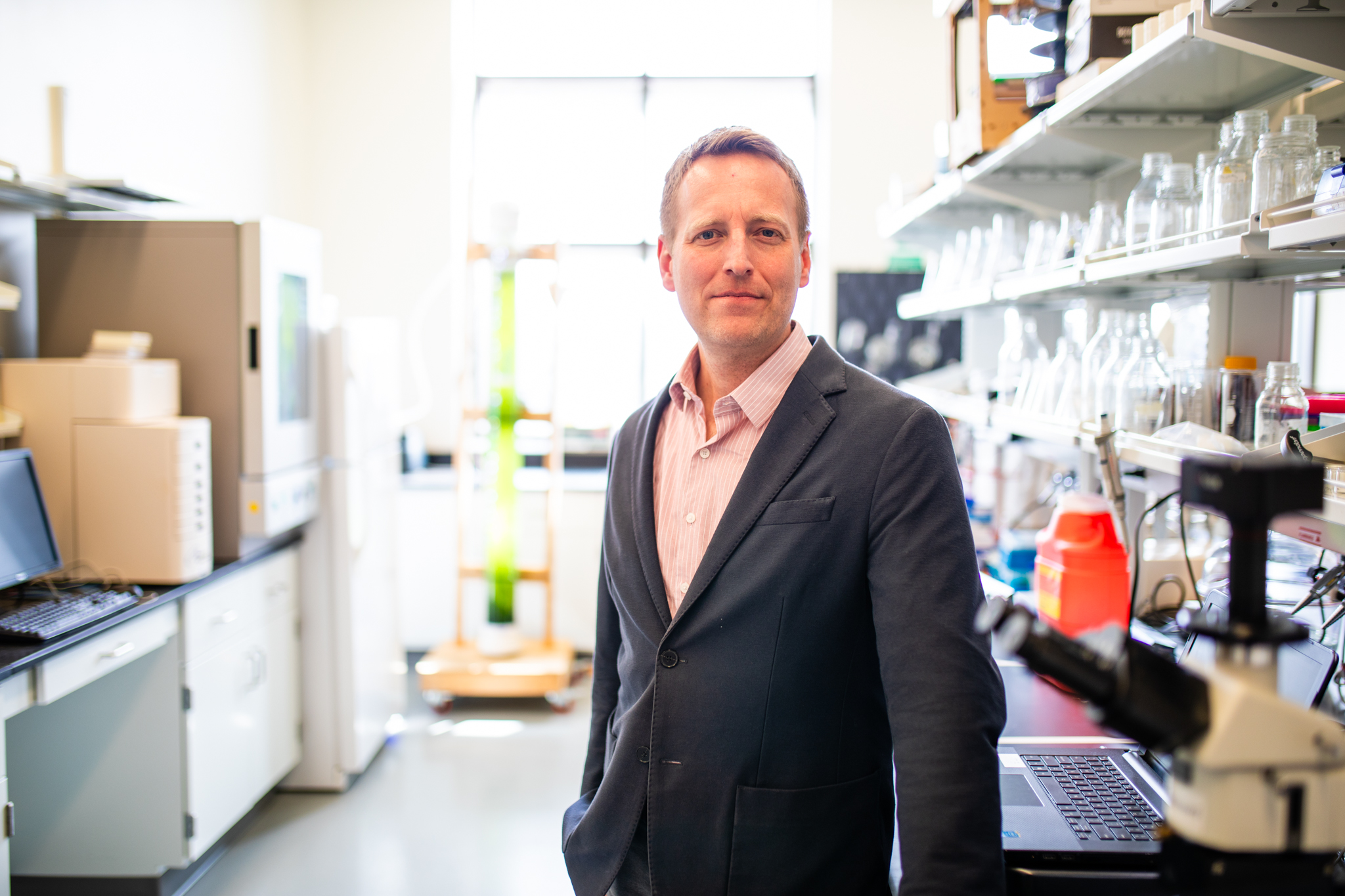
What’s up with the kidney?
RS: Recently, the epidemic of CKD has increased. The number of incidents has grown tenfold in the last 30 years or so, with the risk factors of diabetes and high blood pressure increasing in levels as well. By identifying the onset of CKD earlier, a patient can make lifestyle changes that can slow or stop the progression of the disease. However, it can be very rare to find CKD in its early stages, as symptoms are not usually present until the disease has progressed. At that point, the kidney damage is irreversible. So, it’s easy to see how early detection helps not only doctors, but patients as well.
Why did the doctor call the engineer?
RS: Our field is the area of disease detection. By detecting diseases earlier and accurately, you’re able to help the patient. The hard part is creating a test that can detect biomarker molecules and metabolic profiles at very low concentrations. As engineers that's what we're trying to do - increase sensitivity, but also engineer and increase specificity to the disease. Most current tests only test for diseases and ailments that are already fully present within the body - typically when the disease has progressed to a later stage. Through Raman spectroscopy, detection can happen sooner, as disease screening can occur more regularly and is non-invasive. By working alongside doctors, engineers can understand the human perspective and response to the disease at various stages. Engineers can use this experience to design the latest technology that will detect and advance our knowledge of diseases. In the case of Raman spectroscopy, we have done this by using the unique Raman molecular fingerprint of urine. This has resulted in a test that is inexpensive, rapid, and avoids blood-draws. Nothing will replace the expertise of physicians to diagnose disease and design treatments, but engineering new tests and tools improves patient outcomes and their experiences.
Ideally, in 10 years…
RS: This potential for rapid screening tests will help detect diseases earlier, allowing for physicians to start treatments sooner or run additional diagnostic tests. Ideally, rapid screening tests would become part of routine check-ups at your doctor visits, especially when it comes to kidney and bladder health. Our Raman-based rapid screening tests have the potential to lead to the detection of other diseases, and we have seen success in detecting acute and long COVID-19, Lyme disease, and microhematuria, among others.
Wei Zhou
Associate professor in the Bradley Department of Electrical and Computer Engineering
For nearly a decade, Wei Zhou has stood at the intersection of health and electrical engineering. Zhou’s research centers on an interdisciplinary approach to nanophotonics – the study of light – and nanoelectronics – incredibly tiny electronic devices. He uses this combination of lasers and electronics to create microscopic devices that go inside cells to read their biochemical and electrical activities.
Wearable biosensors are commonly used in healthcare applications to monitor a patient's bodily responses and biological functions, such as for cardiac disease or diabetes. The sensors provide a noninvasive way to deliver the patient's information quickly. However, the future of biosensors, according to researchers like Zhou, are microscopic electrodes. From inside the cells, they can retrieve ongoing information about the body’s functions, all without the cell even realizing it’s there. It’s like having a tiny doctor in the patient's body, helping monitor, diagnose and treat each individual medical circumstance.
In Zhou’s words…
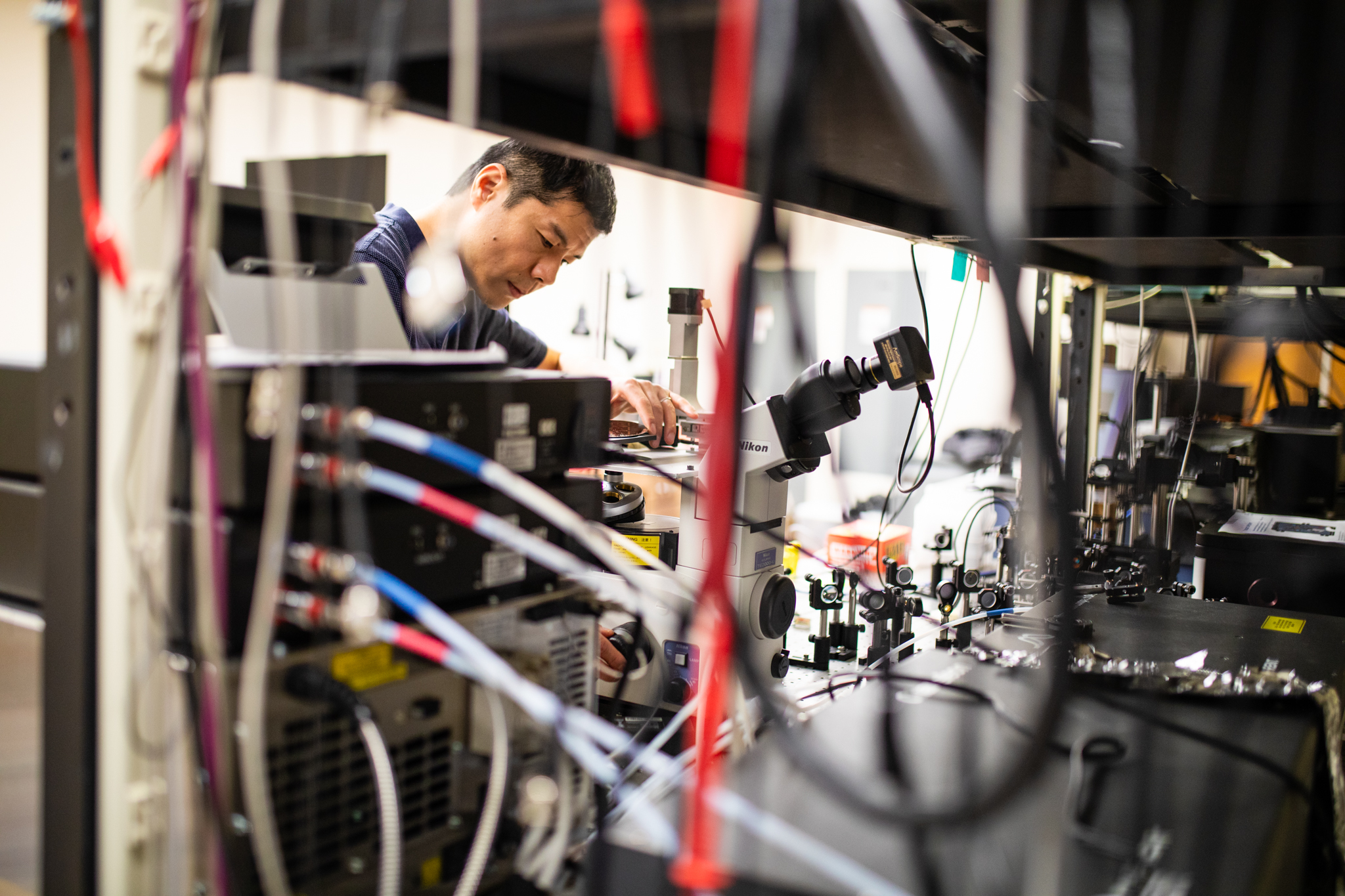
What’s up with the human cell?
WZ: The living, human system is the most complicated system, unique to every person on the planet. Within our bodies lies a universe of information about what we need to nourish ourselves, when we should rest for healing, and deep down, how our body is affected by internal activities. Current diagnostic tools that access cell data – like a biopsy – remove the cells from their “home” system and kill them to retrieve the information. At that point, the cells are rendered useless.
But what if the cells could remain living? My research injects nano-optoelectrodes into cells within the human body and the complicated systems inside us share continuous, real-time data – data for diagnoses and personalized treatment.
Why did the doctor call the engineer?
WZ: Much like doctors themselves, nano-optoelectrodes perform “surgery” to go inside human cells. The electrode’s distinctive pillar shape – it actually looks like a tiny Eiffel Tower – tricks the cell into engulfing it. Then, using a short-pulsed laser, the device makes bubbles in the cell’s membrane to create a space for it. The devices use machine learning to process and send out the intracellular activities, just like getting any test in a hospital. From a CT-scan or an MRI, or even a simple blood test, doctors analyze the results to get a diagnosis – whether you’re healthy or not. These nano-electrodes do the same thing, just from inside your cells.
Ideally, in 10 years…
WZ: The next frontier of health care is truly knowing an individual’s body inside and out, with medical interventions that are personalized and specific to the patient. Beyond high-contrast dyes, or implanted glucose-monitoring devices, nano-electrodes from inside the living cell will provide the real-time, intracellular network data doctors have never before been able to access. At this level, doctors will not only understand their patient, but also the intricacies of their illnesses and the effects of drug therapies.
Glucose-monitoring devices, for example, measure one specific part of a person’s body. In that case, their blood sugar levels. But with a tiny team of microscopic devices, we can study a patient’s brain signals, how cells are working to fix an injury like a cut or fight an infection, and even monitor blood sugar levels – all at the same time. Wherever an electrode is injected, we can gather data.
Since the first injury, physicians have pursued the best patient outcomes – researching, conducting clinical trials, pushing themselves through years of training, and hours of surgeries. With the help of a nano-photonics engineer, treating the whole patient quickly, efficiently, and correctly will become much easier.
By Florence Gonsalves, Briana Bittner, Michelle Darby, Niki Hazuda, Tonia Moxley, and Hailey Wade.
If you want to have an impact on our students and faculty like those featured in this magazine, go here to support the College of Engineering. For more information, call (540) 231-3628.
-
Article Item
-
Article Item
-
Article Item